RESEARCHING VENOM can be a hazardous pursuit. Ask Associate Professor Bryan Fry. This Brisbane-based molecular biologist has been bitten 27 times by venomous creatures, mostly snakes on land and box jellyfish and stingrays at sea. He’s also had 23 broken bones and 400 stitches, has been concussed three times and once fractured his spine in three places, after which he spent months in hospital relearning how to walk.
Bryan is no masochist. But if you study venom, you need to go into some wild places and confront a lot of deadly creatures to collect the material that’s fundamental to your research. It may be dangerous, but it’s necessary, because Bryan’s work with the venoms he collects is crucial to making the antidotes – the antivenoms – needed to treat the millions of people bitten around the world each year by venomous animals.
Of course, there’s no one antivenom that works across all species: each needs to match the toxins of a particular species. To make matters more complicated, the toxins can vary widely within the same species, depending on the environment they live in and the prey they take. Without a detailed understanding of exactly what’s in a venom, it’s not possible to predict how the human body will react to it, what organs will be affected and how to treat a patient.
“There is a global database of antivenoms maintained by the World Health Organization, but it’s based on what is known about each species of snake,” explains Bryan, who heads the Venom Evolution Lab at the University of Queensland (UQ). “How well these have been tested against the full geographic range of any particular snake, or how it performs against snakes that are close relatives and may therefore have some cross-reactivity, we just don’t know.”
Much of the lab’s time is taken up delving into the extraordinary complexity of venoms. And that means so much more than just determining what toxins make up the venoms of the world’s venomous creatures. It’s often also about exploring the sometimes-sizeable toxin variability between geographic ranges of the same species. And it can be about investigating what elements of one antivenom might work across related or unrelated species – known as cross-reactivity.
To do all this, the lab’s researchers rely on the world’s most diverse venom collection – ranging from Antarctic octopuses, king cobras and Komodo dragons to vampire bats – much of it collected during Bryan’s two decades of fieldwork.
Understanding venoms is essential, because envenomation – being bitten by a venomous animal – is a huge global problem. Around the world about 5.4 million people a year are bitten by venomous snakes alone. Between 81,000 and 138,000 of them die. Venoms not only kill but can cause paralysis, bleeding disorders, kidney failure and tissue damage, all of which can lead to limb amputation or other permanent disabilities. Children suffer more severely because of their smaller body mass.
As one of the world’s leading venom specialists, Bryan is an author on almost 250 scientific papers and has led 40 field expeditions to collect venoms. And he’s contacted by people from all over the world, sometimes in the middle of the night, asking for help.
“I get phone calls at two in the morning from researchers who are assisting doctors to treat bite victims,” he says. “I recently consulted on a bite in Brazil, of somebody who kept a pet cobra illegally, and one veteran researcher asked, ‘What’s this cobra’s venom likely to do?’ It was one we’d worked on, so I said, okay, in addition to the paralysis, for which they’ll need artificial respiration, this cobra’s venom also affects the muscles. So you need to monitor for muscle breakdown…and [possible] kidney failure.”
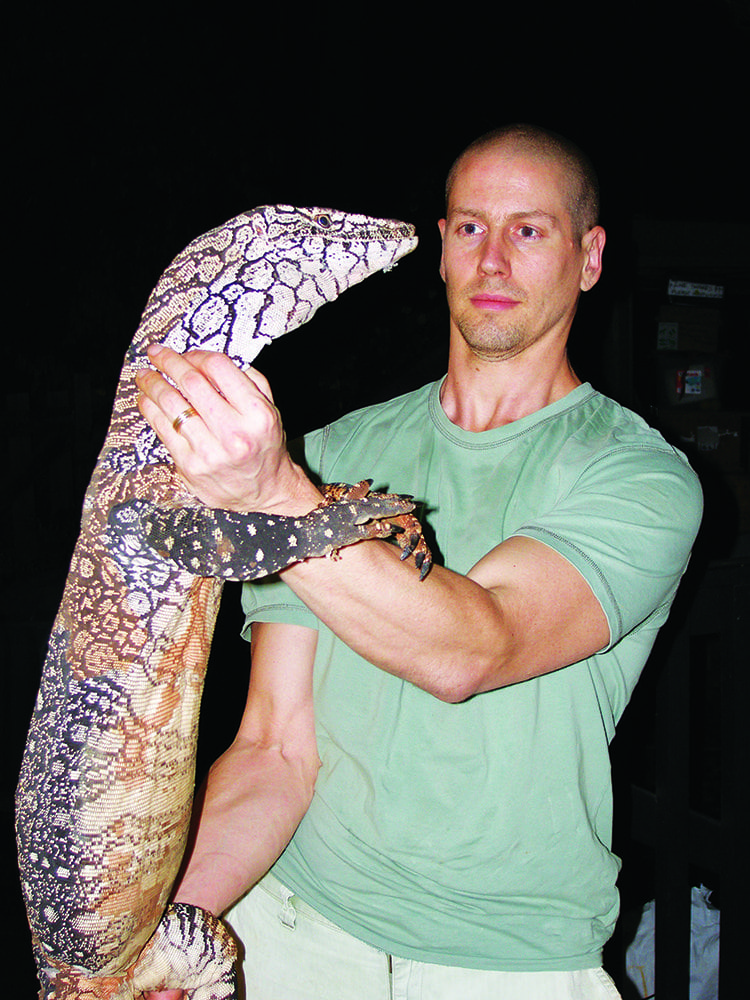
biggest goanna. (Image credit: Museums Victoria)
Welcome to the Venom Evolution Lab
Venom is becoming increasingly attractive to medical researchers and it’s easy to see why.
“One of the most diverse, versatile, sophisticated and deadly biological adaptations ever to have evolved on the planet,” is how biologists Ronald Jenner and Eivind Undheim describe it in their book Venom: The Secrets of Nature’s Deadliest Weapon.
“A venom,” they write, “acts more like a battalion of snipers than a machine-gun loaded with one type of bullet. Because the most complex venoms contain hundreds or even thousands of distinct components, they are able to overcome the defences of almost any victim. Moreover, venom toxins act as self-guided bullets.”
This sort of complexity makes venom attractive to scientists seeking to better understand how the human body works. They can use it to tweak the body’s internal machinery to correct problems, fight disease and improve health.
That’s because our bodies, like those of most animals, have an astonishing galaxy of components – from multitudes of proteins and enzymes, fatty acids such as lipids, vitamins, salts such as sodium and potassium, trace elements and signalling molecules that all interact with each other, under the control of genes. And all of this works in mostly unknown or little-understood ways.
Having evolved during millions of years to alter or subvert the molecular machinery of so many species – including humans, either deliberately or accidentally – and in a dizzying array of pathways, venoms represent a treasure trove of potential insights into the ways our bodies work.
You can clearly see that potential being exploited in Brisbane at one of the world’s pre-eminent centres for venom research. It’s a four-minute walk from the concrete-and-brick Gehrmann Laboratories where Bryan’s Venom Evolution Lab is housed, to the sweeping curves of glass, steel and sandstone of the Queensland Bioscience Precinct.
In the midst of this $130 million showpiece, completed in 2002, stands the palm-fringed Institute for Molecular Bioscience. Between them, these two locations have some of the most sensitive, fancy and expensive scientific instrumentation in Australia, much of which is designed to coax insights from the tiniest fragments of molecules.
At the institute there are 21 high-tech microscopy facilities, and an auditorium-sized mass spectrometer able to do 100 scans per second to a resolution of less than one part per million, identify 1200 proteins an hour and map the plethora of peptides in a venom. All of this is backed by high-powered graphics-accelerated computers for image processing and visualisation.
In this hive of 21st-century scientific bravura, some 400 scientists probe the genetic and molecular basis of living things, seeking to apply their findings to the design of new drugs or the development of new treatments that improve health, combat disease or make cities and food more sustainable.
“This is the centre of the world in terms of venom research,” says Professor Glenn King, a biochemist at the institute. “There are other places doing excellent work in antivenom research, and groups in Belgium and Melbourne doing great work on venom toxins, but in terms of research breadth across drug development, pharmacology, chemistry and structural biology, we’re definitely the leaders.”
It was Glenn’s interest in insects while at Sydney’s selective Hurlstone Agricultural High School that brought him into the venom field. Later, studying insecticides at the University of Sydney in 1995, he was asked by a colleague at Deakin University in Geelong, the late Dr Merlin Howden, to look at the structure of an interesting molecule in funnel-web spider venom, which eventually led to a paper in Nature Structural Biology.
“I thought, ‘Wow, this spider stuff is cool’,” Glenn recalls. “I asked [Merlin] for venom to look at it in more detail. He said, ‘Sure, I’ll send you some.’ Next thing I know I get glass pipettes with freeze-dried venom in the mail. It’s hilarious. You couldn’t do that today.”
Glenn used liquid chromatography to separate out the venom’s different compounds. He was expecting the machine to spit out two or three peaks, from which he would discern the active peptides – short strings of amino acids that carry out essential biological functions.
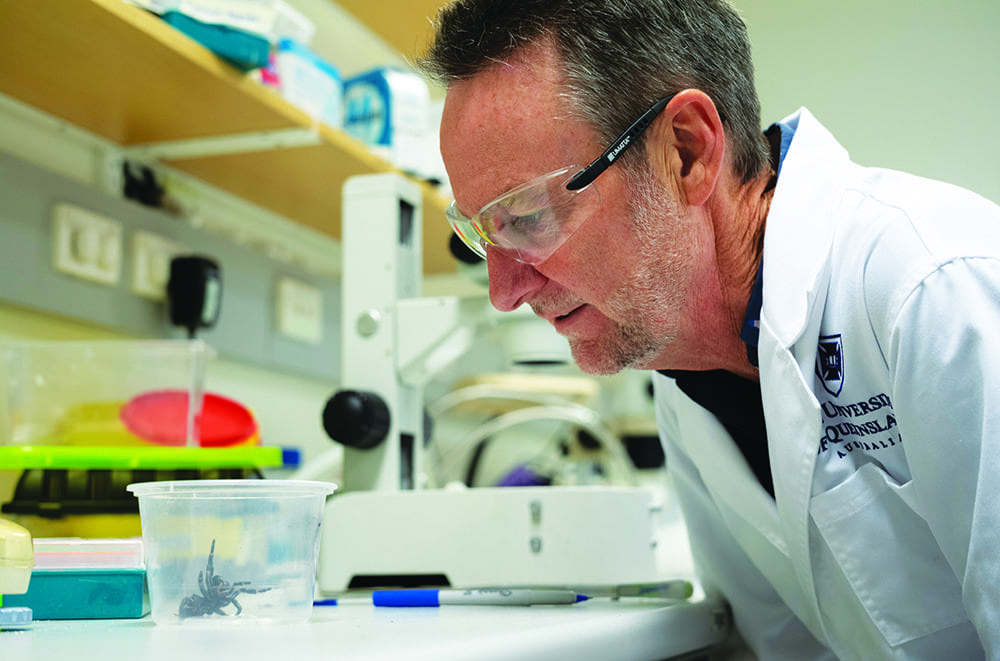
“But the peaks just kept coming and coming – there were hundreds of compounds,” he says. “And I thought, ‘My goodness, this is all completely uncharacterised, nobody knows what most of this stuff is.’ We now know the venom of the funnel-web alone contains more than 3000 peptides. We think it might be the most complicated chemical arsenal in the natural world.”
That diversity is found across venoms, says his colleague Associate Professor Irina Vetter, co-director of the Centre for Pain Research at the institute.
“Nature has provided us with this amazing library of millions and millions of compounds, and they all do something,” she says. “And if you’re looking for tools to help you understand how sensory nerves function, or how to affect them, venom is just a treasure.
“Sometimes it’s really unexpected where it can take you. You can actually learn a lot about how pain-sensing nerves work, how the channels are activated, and new ways to modulate them – and hopefully create new treatments.”
Venoms have already given the world six new drugs, one of which is Ziconotide, derived from a species of marine cone snail and which is highly effective against severe chronic pain. Another nine are presently undergoing clinical trials. Irina says that while Ziconotide proves venom-derived peptide painkillers are possible, it has to be injected into spinal fluid, “which is less than perfect”. Her team hopes to develop easier-to-use painkillers that could be taken once a week or less.
An area of the institute’s focus are the nine different sodium channels in humans – multi-layered biological switches that create and respond to the body’s electrical signals. They are implicated in a variety of diseases including epilepsy, irregular heartbeat and nerve pain caused by trauma, surgery, disease or chemotherapy. One subtype is predominantly in the heart, another almost exclusively in skeletal muscle, and three subtypes are only found in pain-sensing nerves. But Irina’s team has discovered, thanks to scorpion venom, that a subtype for touch sensing also plays a part in pain.
“In pain research, we’re at a stage where cancer research was maybe 30 years ago, where people started to realise, ‘It’s actually way more complicated than we thought’,” Irina says. “If you have cancer, I can chop out a bit of the cancer and study it. If you have pain, I can’t really chop out your pain-sensing nerves to understand what’s going on. So it’s really difficult to correlate pain you’re describing to a molecular mechanism.”
In an underground room behind a double steel door is an insectary where some 100 rectangular plastic trays and round takeaway food containers are each filled with a little soil and a single spider. There are various species of huntsman, tarantula, wolf, trapdoor, funnel-web and mouse spiders – just some of the world’s 50,000 named arachnid species – and a collection of venomous centipedes and scorpions.
Samantha Nixon, a PhD student of Glenn’s, shows me how she milks venom for research. She places a small container with a single Sydney funnel-web at the centre of a larger tub. Taking a long pipette with a thin, hollow extender, she gently pokes the spider around the head, triggering its attack mode, and then swiftly drains its venom gland with one squeeze. Not bad for someone who, she admits, was once an arachnophobe.
It’s not just spiders, snakes and cone snails that yield venom. Researchers are exploring plants too. Back in Irina’s office, a fuzzy-leafed Gympie-Gympie sapling is perched by the window. Irina picks it up to show me the dense hairs on the surface of the leaves, which, if touched, act like hypodermic needles to inject a potent neurotoxin.
In a study recently published in the journal Science Advances, her team reports on the discovery of toxins that had only been seen before in the venom of spiders and scorpions, although their chemical composition is very different. They cause pain in the same way – by modulating sodium channels in pain-sensing nerves – but do so in a way that makes pain that can last for days or even weeks.
Why would a plant evolve a neurotoxin? Irina has no idea. But being a pain researcher means she’s interested in the mechanisms of pain and how we feel it, so she can develop new treatments. That occasionally means being on the receiving end, as she has been with the Gympie-Gympie: “You get redness and weals, it starts to burn, the pain becomes wavelike and can radiate to your lymph nodes, and you get a deep aching sensation in the shoulders,” she recalls. “It’s very strange.”
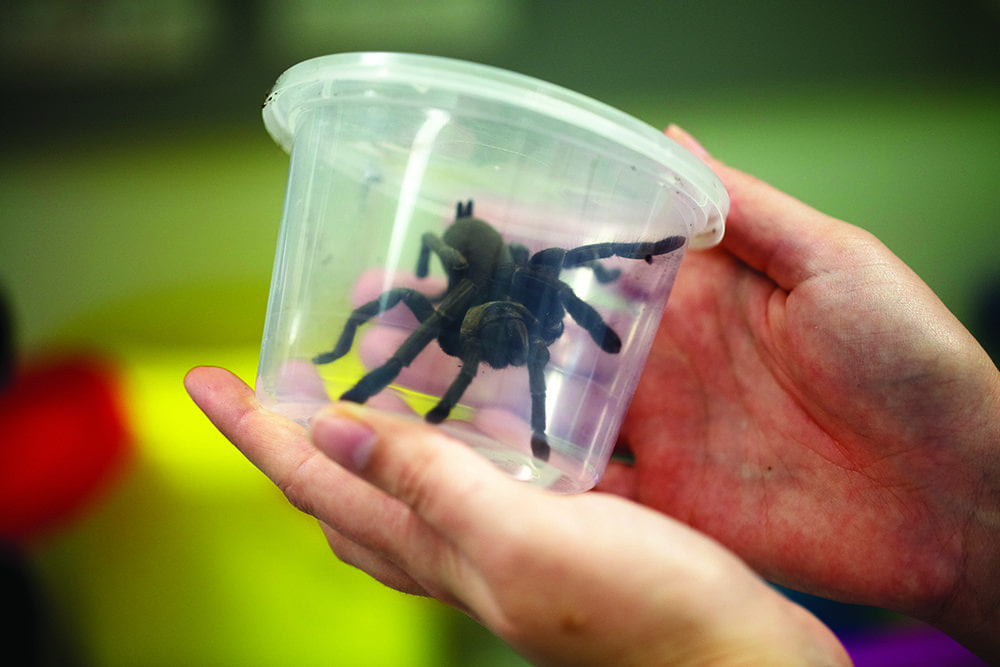
The beginnings of a passion
Bryan Fry came to UQ for his PhD after completing undergraduate degrees in Oregon, in the USA. Fellowships in Singapore and Melbourne followed before he returned to Brisbane to head up his own lab.
The place buzzes with bright doctoral students from all over the globe and is brimming with a panoply of high-tech equipment such as the world’s fastest, most reliable, blood-clotting analyser. Christened Dracula, it’s the only machine of its type in Australia.
Then there is a massive glass cube housing a robot with three arms. It’s the heart of a $2 million biosensor facility – the only one in the Southern Hemisphere – that measures the binding of molecules down to the size of two hydrogen atoms. That one’s dubbed Skynet, after the giant super-intelligent computer network in the Terminator movies.
Bryan recalls being in an isolation ward at the age of two, fighting the bacterial toxins of spinal meningitis, which can cause brain damage, paralysis and stroke. He had to be strapped down to a hospital bed because, in distress, he’d try to rip out the surgically implanted tubes in his temple and ankles that delivered medicine.
“That’s my first memory – being torn apart by toxins,” he recalls. “I remember my little blue blankie and my yellow rubber duckie we brought to hospital. They took those away and incinerated them.” Bryan survived, but lost hearing in his right ear and still has an impaired sense of balance.
It triggered a life-long interest in toxins of all types, what they do and how they do it, and that morphed into a fascination for venomous snakes. “I was four when I told my parents that I was going to make the study of venomous snakes my life’s work,” he says. “I managed to turn that childhood passion into a career.”
It’s taken him across the globe searching for venomous creatures. Now married and with a new baby, Bryan eschews what he dubs his “crazy pre-marriage past”– or what his wife, Kristina, calls “travelling to far-off places trying to get himself killed in unusual ways”.
At the lab he also maintains a ‘pickled zoo’ of preserved venomous animals and uses a 3D-printer to make replicas of their skulls. “We’re interested in the venom glands,” Bryan explains. “With these prints, we can look at changes in skull morphology and relate that to changes in the venom delivery apparatus and the venom itself, because it’s all an integrated weapons system.”
For exotic venoms, Bryan still occasionally mounts an expedition but now mostly depends on his network of fellow researchers and their forays into the wild. For the more common venoms he relies on his lab manager, Dr Christina Zdenek, a postdoctoral research fellow and a former PhD student of his who happens to keep some of Australia’s deadliest snakes in her home.
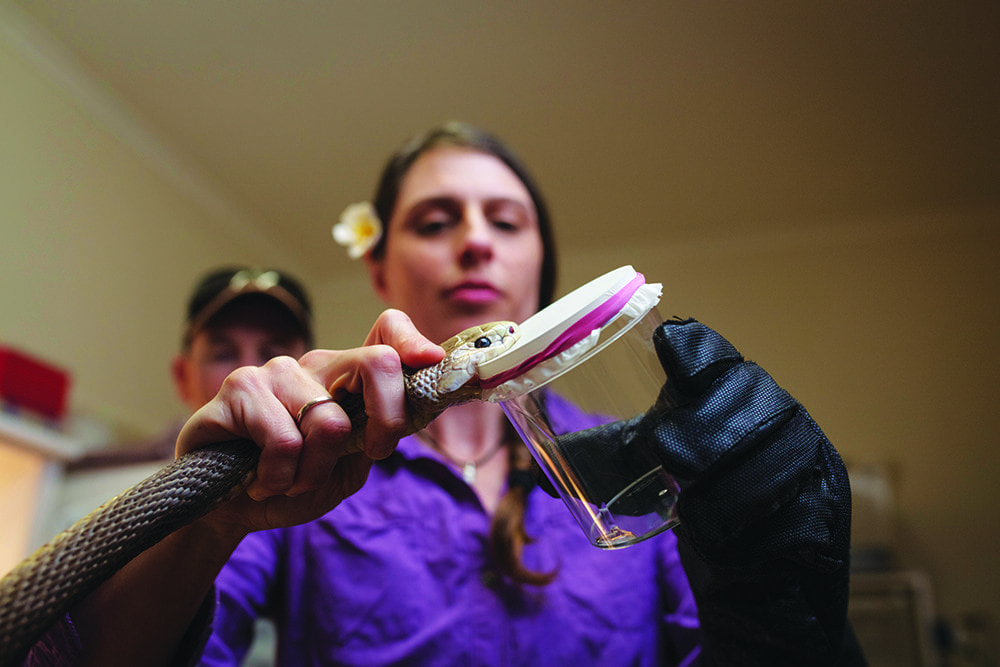
At the suburban townhouse she shares with her husband, Chris Hay – himself a licensed snake demonstrator turned herpetologist – is a den of 21 mostly highly venomous snakes – coastal taipans, death adders and eastern browns with pet names such as Squishy, El Diablo, Mr Naughty, Lumpy and Casper.
She stoops to a glass cabinet while she watches a coastal taipan intently as she uses a hook to prise it up from its cubicle. She holds it for me to see – left hand on the tail, right hand using the extendable hook to gently hold the snake’s head at a distance.
Also an American, Christina got her undergrad biology degree in California and came to Australia to study palm cockatoos before doing her PhD at UQ on her first love – snakes, specifically the toxicology and conservation of death adders, one of the world’s most venomous land snakes.
Ironically, for a creature armed with a deadly neurotoxic venom, death adders themselves are threatened by cane toads: the amphibian eats young death adders, and adult death adders eating the toads are poisoned by the toxic glands in their skin.
Christina’s loved snakes since she was five, when her brothers got one as a pet. At 11, she began breeding veiled chameleons, lizards native to the Middle East, and grew up with all manner of other reptiles in the house.
“They’re fantastic pets. They’re not noisy, they don’t destroy the furniture and, depending on the species, you can leave them alone for weeks if you’re on holiday,” she explains. “I would study their behaviour so intently as a little girl. I still love getting my hands on snakes and seeing them up close, but I’m careful with the venomous ones.”
Christina is now studying snake venoms, and most of her research papers arise from venom she milks from the snakes at home. She’s found that snake venoms – even within the same species – can vary by sex, age, geography and even local temperature. She’s also studying how well existing antivenoms work, what active compounds they do and don’t neutralise and what elements of one antivenom have cross-reactivity with other venoms.
She hopes the benefits that science and medicine are deriving from venom will allow the public to appreciate that snakes and other venomous animals not only perform essential roles in nature but are valuable to society.
“Venom is very common throughout the tree of life,” Christina says. “You’ve got tens, even hundreds, of millions of years of evolution, which has honed these very special cocktails to enable each species to survive and thrive, that transforms capturing prey from a physical battle into biological warfare. And that’s turned out to be a goldmine for us humans.”